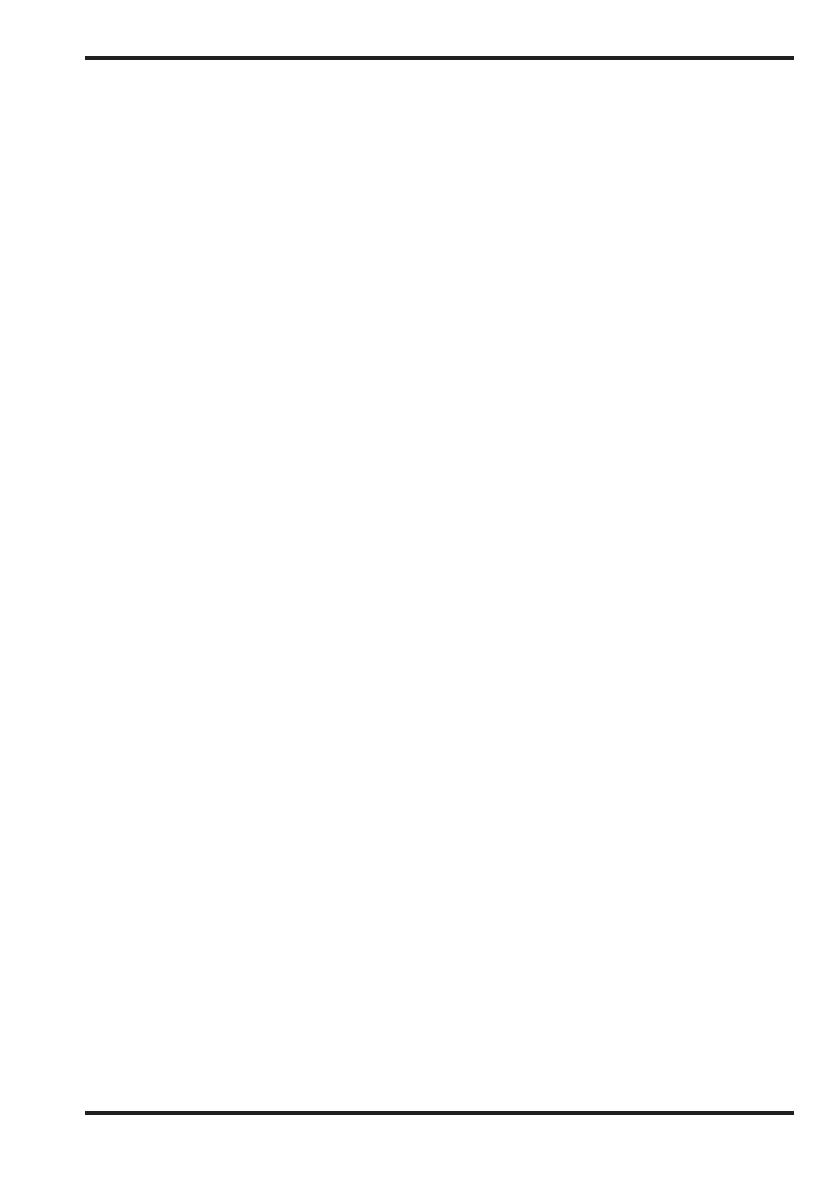
Änderungen vorbehalten / Reservado el derecho de modificación 23
Table of contents
Operating instruction . ...............................................................24
Near Field Sniffer Probes HZ 530 ................................................. 24
Specifications ................................................................................ 24
General Information ....................................................................... 26
Safety ............................................................................................. 26
Operating Conditions ..................................................................... 27
Warranty ........................................................................................ 27
Introduction ................................................................................... 28
Operation of the Probes ................................................................29
Battery Operation .......................................................................... 29
Output Impedance Matching ........................................................29
Use of different probe types ......................................................... 30
Safety Notice ................................................................................. 30
Accuracy Notice............................................................................. 31
Basis for Near-Field Probe Measurements ...................................31
The H-Field Near-Field Probe ........................................................31
The High-Impedance Probe ........................................................... 32
The E-Field Monopole Probe ......................................................... 32
HZ 530 Near-Field Probe Applications ..........................................33
Practical Selection of Signal-Line Filters ....................................... 33
Measurement of the Shielding Attenuation of ............................. 39
Shielded Housings with the E-Field Probe .................................. 39
Commonly asked questions about
pre-compliance emissions testing . .......................................... 41
How can pre-compliance instruments be defined? ................ 42
Why is there such a cost difference between ....................... 42
compliance and pre-compliance test instruments? ................ 42
How important is EMC training? ............................................. 43
How important is an instrument’s amplitude accuracy? ........ 43
Should pre-compliance instruments
contain “CISPR bandwidths”? ................................................ 43
How will the level of ambient signals affect my
radiated emissions measurements and will using fully
compliant measuring equipment help? ................................... 43
Will a screened room overcome
the problem of ambient signals?.............................................44
Can I make meaningful radiated emissions measurements
with a near field probe instead of an antenna? ...................... 44
Do spectrum analyzers have any
advantages over receivers? .................................................... 45
Will a spectrum analyzer allow me to make
sensible emissions measurements?....................................... 45
Does a spectrum analyzer’s response to pulsed
interference influence the measurement result? ................... 45
What are the results if I surround the entire
equipment under test in a shielded housing?......................... 46
Does the E-Field-Monopole of the HZ530 have
sufficient sensitivity for pre-compliance testing? ................... 46